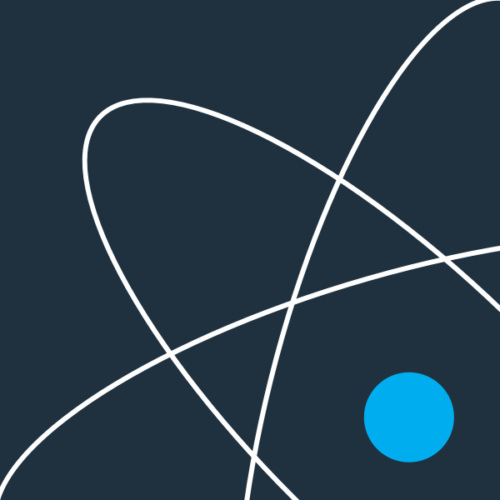
Anya Loukianova
Research Associate, The James Martin Center for Nonproliferation Studies
Every year, medical professionals worldwide carry out more than 30 million procedures using the medical isotope technetium-99m (Tc-99m), over half of these in the United States.[1] A radioisotope that decays over six hours, Tc-99m is injected into the human body to assess the presence and progress of ailments such as heart disease and cancer.[2] In a hospital setting, Tc-99m is derived from special generators that incorporate its parent, molybdenum-99 (Mo-99).[3] But because Mo-99 has a relatively short half-life of 66 hours, these generators cannot be stockpiled and must be replaced on a weekly basis.
The United States does not produce commercial quantities of Mo-99. Most of the Mo-99 supplied to the domestic market is produced abroad through fission using highly-enriched uranium (HEU) in a handful of research and test reactors. These foreign reactors (or, irradiators) are powered by HEU fuel and/or irradiate HEU targets, and acquire the several dozen kilograms of HEU required from either the United States or Russia. The facilities that process the targets after irradiation in order to extract Mo-99 (or, processors) also house waste containing HEU materials.
For over three decades, U.S. policy has aimed to minimize the use of HEU in the civilian sphere because of proliferation and nuclear terrorism concerns. The U.S. government has worked with international partners to convert research reactors from HEU to low enriched uranium (LEU), shut down HEU-powered facilities, and secure HEU during transport, processing, and storage.[4] More recently, the U.S. government has advocated replacing aging reactors that use HEU for Mo-99 production with new facilities that utilize LEU or alternative Mo-99 production methods.
Between 2005 and 2010, lengthy irradiator outages and several other incidents caused severe shortages of Mo-99 for medical procedures worldwide, demonstrating the fragility of the radioisotope's supply chain. Moreover, a Canadian and a European reactor that have been mainstays of the medical isotope production fleet intend to shut down within the next decade. Meanwhile, the global demand for Mo-99 for medical procedures is projected to continue to rise.
This brief discusses the evolving structure of the Mo-99 market and assesses executive, legislative, and international efforts to balance the trade-offs between security of medical isotope supply and nuclear security.[5]
Mo-99 as "The Queen Mother"[6]
Discovered by two scientists at Lawrence Berkley National Laboratory in 1938, Tc-99m is today the most widely used radioisotope in nuclear medicine.[7] Introduced into the body of a patient, Tc-99m emits energy that can be observed through special cameras and then rapidly decays, leaving no trace of its presence.[8] The demand for Tc-99m, vital for non-invasive diagnostic procedures, is projected to steadily rise through 2030.[9]
The use of Tc-99m became widespread in the 1960s, and initially, research and test reactors at U.S. national laboratories and universities provided enough Mo-99 to satisfy domestic demand. The Atomic Energy Commission, a predecessor of the Department of Energy (DOE), produced Mo-99 at Brookhaven and Oak Ridge National Laboratories. The University of Missouri Research Reactor (MURR) produced Mo-99 on a smaller scale starting in 1967.[10] When demand outstripped supply, private industry stepped in to both produce and distribute Mo-99.[11]
Private industry was the first to use HEU for Mo-99 production; earlier producers had relied on neutron absorption in molybdenum targets. In 1980, Cintichem, Inc. began to produce the medical isotope through neutron-induced fission reactions with HEU targets.[12] This process allowed for more efficient recovery of Mo-99 than the previous techniques. However, the Cintichem, Inc. reactor in Tuxedo, New York was forced to shut down in 1989 due to tritium contamination concerns, ending U.S. Mo-99 production.[13]
In the 1990s, private industry in the United States "was not willing to assume the financial and regulatory risks associated with building and operating a new reactor facility."[14] Cintichem, Inc. instead arranged for a Canadian company, Nordion, to supply Tc-99m generators to the U.S. market.[15] The Mo-99 for these generators was produced at the Chalk River facility's NRU reactor that utilized HEU for both fuel (through 1993, when it converted to LEU fuel) and targets.[16]
In response to security of supply worries by U.S. isotope users, the DOE first purchased the Cintichem, Inc. technology and then initiated studies of several potential Mo-99 irradiators, including reactors at Los Alamos and Sandia National Laboratories.[17] Despite the conversion of a facility at Sandia for this purpose in 1999, the domestic production of Mo-99 was never initiated.[18] The DOE also briefly funded a joint U.S.-Russian study on Mo-99 production that envisioned that U.S.-based company Technology Commercialization International (TCI) Medical would cooperate with Russia's Kurchatov Institute on developing an alternative production method using an aqueous homogenous reactor (AHR) fueled with uranyl nitrate for Mo-99 production.[19] Despite all of these efforts, the United States firmly relied on foreign producers, all using HEU, to supply its domestic Mo-99 needs by the end of the 1990s.[20]
Got Tc-99m?[21]
The weekly delivery of Tc-99m/Mo-99 generators to hospitals hinges on the continuous operation of irradiators and processors, and a complex supply chain that relies on express shipments of radioactive cargo across borders.[22] The shipments to and within the United States are carried out by passenger and cargo aircraft (and, less frequently, trucks).[23] All aspects of these deliveries, from packaging to the carrier's transport routes, are guided by national and international regulations.[24]
The Mo-99 production process begins with the advance supply of HEU fuel and targets to the irradiators (and target manufacturers) abroad. The United States and, less frequently, Russia have been the major suppliers of this HEU. Annually, approximately 45 kilograms of HEU are used up in Mo-99 production.[25]
Mo-99 production process
Source: TRIUMF, inspired by graphics from Nordion
A research and test reactor irradiates the targets for approximately seven days.[26] The neutrons in the reactor bombard the targets, causing the split of U-235 atoms. The irradiated targets are then cooled and rushed to a processing facility. In hot cells at this facility the targets are dissolved and the Mo-99 is recovered, then subsequently purified and made into a bulk Mo-99 solution. This processing takes up to a day to complete.[27]
Because of the targets' relatively short irradiation time, more than 90% of the HEU remains in target waste after the completion of Mo-99 production. This waste is not considered to be "self-protecting" after a certain cooling period, which means that a would-be thief could handle it without risking immediate incapacitation.[28] Target waste could also be converted into HEU metal to produce a gun-type nuclear explosive device.[29] Because of this "proliferation-sensitivity," the International Atomic Energy Agency (IAEA) outlines secure storage procedures for this waste.
After processing, the bulk Mo-99 is sent to companies that manufacture the Tc-99m/Mo-99 generators. The generators, which are essentially shielded cartridges containing Mo-99 solution adsorbed into an alumina column, are then rushed to pharmacies and hospitals.[30] This urgency is necessary because the activity of Mo-99 begins to decline from the point at which irradiated targets are removed from a reactor and continues its decrease through processing.[31] Upon receiving the generator, a hospital can extract Tc-99m from the generator for about a week by passing saline through the alumina column.[32]
Like any complex network, the production, processing, and delivery of Mo-99 may fail to work as planned. Between 2005 and 2010, a product recall at a major generator producer and lengthy reactor outages caused severe shortages of Mo-99 for medical procedures in the United States and elsewhere. These outages had a dramatic impact on the Mo-99 supply chain and have spurred the U.S. government and the international community into action.
The U.S. Mo-99 Supply Chain
Through 2010, the U.S. supply chain's peculiar structure included five major reactors, four major processors, and two generator manufacturers. The irradiators, all using HEU targets and some also using HEU fuel, were spread across three different continents.[33] They included Canada's NRU, Belgium's BR-2, France's OSIRIS, the HRF in the Netherlands, and South Africa's SAFARI. The processors included Canada's MDS Nordion, Belgium's IRE, Covidien (then known as Mallinckrodt) in the Netherlands, and South Africa's NTP. The international companies Covidien (Mallinckrodt) and Lantheus manufactured the Tc-99m/Mo-99 generators and supplied them to hospitals.
The cascading failures in the supply chain began in 2005. That year, Mallinckrodt halted its production of generators due to a product recall, triggering a shortage that lasted through April 2006. In November 2007, the NRU shut down for over a month and the HFR followed with an extensive outage between August 2008 and February 2009. During this time, an IRE processing facility was also shut down briefly because of an industrial accident. The worst of the outages, however, began when Canada's NRU shut down in January 2009. This shutdown took place through August 2010 and coincided with maintenance of the HRF.
Combined, these events triggered massive supply disruptions, initially forcing hospitals to ration care and cancel procedures. Eventually, hospitals found ways to cope with the shortages by scheduling procedures more efficiently, reducing patient doses of Tc-99m, and increasing the use of alternative imaging modalities such as PET.[34]
Current U.S. Mo-99 supply chain[64]
Producers also began to better coordinate supplies. For example, when one reactor shut down for maintenance, its orders were filled by others. Additional irradiator capacity also came online. Of the eight large-scale irradiators currently online, three are newcomers: Poland's MARIA reactor (currently converting to LEU and utilizes HEU targets processed by Covidien); the LVR-15 reactor in the Czech Republic (recently converted to LEU fuel and uses HEU targets also processed by Covidien); and Australia's OPAL (uses LEU for both fuel and targets, and relies on target processing provided by ANSTO).
However, these new irradiators offer only a short-term solution. The NRU and the OSIRIS reactors are expected to shut down within the next five years. The need to deal with the possible fallout of these pending closures has forced the United States to actively support the establishment of new Mo-99 producers, efforts in turn tailored to support the longstanding U.S. goal of civilian HEU minimization.
Tectonic Shifts
Since 1978, the United States has worked to minimize the amount of HEU in civilian use. These efforts have included the conversion of HEU-powered research and test reactors to low-enriched uranium (LEU), the repatriation of fresh HEU fuel and irradiated HEU in waste, the consolidation of HEU at fewer sites, and security improvements to facilities housing these materials. And, for over two decades, there was some focus on the conversion of LEU targets in Mo-99, a complicated project because of differences in target design and processing techniques worldwide.[35]
In 1992, Congress passed the Schumer Amendment to curb U.S. HEU exports to foreign research reactors, including those for Mo-99 production. This amendment placed several conditions, including a commitment by producers to convert to LEU fuel and targets, on any continued U.S. exports of HEU.[36] But supply security concerns precipitated a shift in Congressional priorities. In 2005, Congress passed the Burr Amendment exempting Canadian and European irradiators from Schumer's strictures and calling for a National Academy of Sciences study to deconflict the two goals. Since then, despite separate actions in both the House and the Senate, Congress has failed to enact further legislation on the Mo-99 issue.
Executive policy has shown greater consistency than Congressional policy. A longstanding DOE National Nuclear Security Administration's (NNSA) program has promoted technical cooperation among reactor operators worldwide, scientists in U.S. national laboratories, and IAEA experts. As part of these efforts (consolidated under the Global Threat Reduction Initiative in 2004), many research and test reactors (including the NRU) converted to the use of LEU fuel, some Mo-99-producing reactors (such as the SAFARI) converted to LEU fuel and targets, and new LEU-based irradiators (such as the OPAL) came online.
In 2009, the National Academy of Sciences finally released the report requested under the Burr Amendment. This study concluded that, there were "no technical reasons that adequate quantities cannot be produced from LEU targets in the future" and that LEU target use was feasible with an acceptable cost increase.[37] The report also recommended that the Mo-99 producers, the DOE, the Department of State, the Food and Drug Administration (FDA), and the U.S. Congress actively move toward the conversion of Mo-99 production away from HEU use.[38]
The NAS report fit well with President Barack Obama's 2009 call to secure all of the world's vulnerable nuclear materials within four years. NNSA accelerated its nuclear security efforts and adopted a two-track strategy for Mo-99 production. The first track promotes the development of sufficient HEU-free indigenous production to supply the U.S. market by 2016, helping to finance the research and development (R&D) stage of four domestic private industry Mo-99 projects. The second track aims to boost foreign HEU-free Mo-99 production and to promote the goal of eliminating HEU-based Mo-99 production through cooperation with international organizations and high-level diplomatic meetings, such as the Nuclear Security Summit.
The Two Track Approach to Mo-99 Production
The official policy of the U.S. government seeks to "end subsidies and establish an economically-sound industry" producing Mo-99 in the United States.[39] Since 2009, the NNSA has supported the development of four private industry projects utilizing a variety of technologies. The financial support for each varies, but is limited to $25 million, and involves a 50/50 cost-sharing agreement with the DOE as well as technical assistance from the U.S. national laboratories.[40]
The NNSA has concluded cooperative agreements with General Electric-Hitachi (GEH); Babcock and Wilcox (B&W); NorthStar Medical Radioisotopes, LLC; and SHINE/Morgridge Institute for Research (MIR), with three of the projects currently underway. In February 2012 GEH suspended its project due to concerns about market conditions, but also noted that it may reevaluate this decision.[41]
Two projects outside the NNSA cooperative agreements, by Coqui RadioPharmaceuticals Corp. and American Medical Isotope Corporation (AMIC), are currently seeking investors and expect to go through the regulatory approval process. (See the following table of domestic producers.)
Table of Potential Irradiators in the United States
The future of all of these projects remains uncertain.[42] Each will need to acquire and sustain funding as its technology and product undergoes the approvals processes of the Nuclear Regulatory Commission and the Food and Drug Administration. Because the projects do not turn a profit until they begin supplying customers with Mo-99, U.S. government support for market entry has been critical. There are, however, medium-term uncertainties about the projects' competitive viability after government subsidies end.
The NNSA has also sought to increase the supply of foreign HEU-free isotopes on the U.S. market until domestic production is established. The NNSA announced in 2010 its financial and regulatory support for a consortium of producers from South Africa and Australia to supply LEU-based Mo-99 to the United States. This consortium has, at times, supplied as much as a third of the Mo-99 market for diagnostic procedures in the United States (when other major reactors have been shut down).[43] The NNSA has also worked with the IAEA to develop small-scale regional production capabilities based on alternative technologies in Eastern Europe, Latin America, and elsewhere, and used its control over HEU supplies to persuade European producers to commit to conversion.[44]
The Nuclear Security Summit process has been critical in promoting action on the challenge of minimizing civilian HEU. At the 2012 Summit, European Mo-99 producers pledged to convert to non-HEU processes in return for continued shipments of U.S. HEU until conversion is completed. In addition, the United States, Belgium, France, and the Netherlands also announced a study to facilitate the development of high-density LEU fuel and target material to make conversion more economical.[45]
Challenges of HEU-free Mo-99 Production
Established HEU-based producers have argued that conversion to the use of LEU targets is uneconomical given existing technologies and could also "leave them at a competitive disadvantage relative to producers who refused to convert."[46] If LEU were simply substituted for HEU in existing HEU target designs, the process would produce less Mo-99 per target.[47] Therefore, producers who converted to LEU have generally had to irradiate and process a much greater number of targets and cope with a much greater volume of waste. Resolving these issues involves substantial up-front investments and, the established producers posit, raises production costs.[48] The newer higher-density targets under development aim to end this discrepancy, but they are not anticipated to become available for commercial use for several years (and likely after the 2015 conversion date currently set for European Mo-99 producers.)
The difficulties faced by established producers pale in comparison, however, with the economic challenges faced by new producers. Established producers use facilities and equipment constructed and purchased by their governments decades ago. Government subsidies for costs related to capital replacement and waste disposition, among others, have enabled established producers to undervalue irradiation services and pass subsidy-derived cost savings along to consumers.[49]
Washington and the OECD's Nuclear Energy Agency have sought to make the market more transparent and competitive by implementing "full cost recovery."[50] If successfully implemented, this approach would force established HEU-based producers to raise their prices in order to account for government subsidies.[51]
Both new and established producers also face regulatory barriers to HEU-free production. When a producer successfully converts to LEU, it faces re-certification costs. For example, the South African producer NECSA has noted that delays in licensing by European and other governments have slowed down its conversion process by two years.[52] The transition to LEU-based Mo-99 must therefore be gradual in order to preserve producers' existing market shares.
A more problematic layer of competition involves new market entrants that intend to use HEU, notably in Russia. The introduction of these actors to the market—before established producers complete conversion and before new market entrants begin production—has the potential to negatively impact the emerging HEU-free Mo-99 industry.
Preferential Treatment of HEU-free Mo-99
Faced with the looming shutdown of its NRU reactor and lacking alternative irradiation capacity in Canada, MDS Nordion has turned to a cooperative venture with Russia's RIAR in order to retain its market share in the United States. This venture, JSC Isotope, plans to utilize HEU fuel and targets for Mo-99 production at the RIAR reactors in Dimitrovgrad.[53] The Russian facility expects to initiate production in 2013 and more than double it by 2015.[54]
In May 2011, U.S. representatives Edward Markey and Jeff Fortenberry expressed concerns in a letter to the DOE regarding the use by MDS Nordion of Russia's HEU to produce Mo-99 for the U.S. market.[55] But these concerns were not followed up with legislative action at that time.
A January 2012 letter to these lawmakers signed by public health and nuclear experts called on Congress to enact a "preferential procurement" clause that would include provisions to "halt[s] the import of HEU-based versions of these isotopes when a sufficient supply of the alternatives is available," a "require[ment for] U.S. health authorities to terminate authorization for use of HEU- based versions when a sufficient supply of the alternatives is available," and the "impos[ition of] a tax on HEU-based versions of these isotopes, channeling any resulting revenue to support production without HEU."[56] But, during an election year, it is unlikely this issue will gain sufficient traction in Congress.
At present, MDS Nordion is completing quality tests of the Russian-sourced Mo-99. In order to enter the U.S. market, this radioisotope still needs to be certified by the U.S. FDA. Recently, Russian officials have noted the possibility of converting to LEU targets by 2018, but they have not committed to converting the targets or the RIAR reactors to LEU or using "full cost recovery."[57]
Meanwhile, the U.S. government is working to make "preferential procurement" a reality. In June 2012, the White House announced that it was committed to eliminating the use of HEU in medical isotopes while assuring the reliability of supply.[58] In order to achieve these goals, official U.S. policy would encourage the purchase of HEU-free Mo-99 at home and abroad, phase out HEU exports when sufficient quantities of non-HEU Mo-99 became available, and continue to support domestic production and foreign producers' conversions.[59]
Washington also called on industry to develop labeling that would allow users to distinguish between LEU- and HEU-based Mo-99 and unveiled other incentives aimed at isotope users.[60] In July 2012, the White House proposed a new Health and Human Services department regulation that would incentivize medical facilities to use HEU-free Mo-99 by paying an additional $10 for each procedure performed on Medicare and Medicaid patients using HEU-freeMo-99. [61]
Conclusion
In June 2012, the Obama Administration announced that "the United States is committed to eliminating the use of HEU in all civilian applications […] because of its direct significance for potential use in nuclear weapons, acts of nuclear terrorism, or other malevolent purposes."[62] Toward this end, focused efforts on eliminating HEU from Mo-99 production are an important step in promoting the security and well-being of publics in the United States and worldwide.
The leadership of key countries, and especially those involved in conversion or new HEU-free production, has been essential in building the emerging HEU-free Mo-99 production consensus. This international commitment could be further strengthened through continued rigorous technical cooperation and high-level dialogues in fora such as the Nuclear Security Summit. If global conversion to LEU is to take place, other countries will also need to use their power as Mo-99 consumers and health regulators to help shape demand for HEU-free radioisotopes through, for example, incentives and speedy licensing processes. Russia's recent hints that it may convert to LEU targets suggest it is reconsidering policy in response to the emerging international consensus against the use of HEU.
Recent radioisotope shortages have highlighted the importance of pursuing nuclear security objectives in a manner that aids supply security rather than undermines it. The role of government incentives for private industry aimed at restructuring the domestic Mo-99 market has been critical to mitigating future shortages. But, it remains to be seen whether one or more of the HEU-free producers will succeed in passing all regulatory hurdles, achieving full cost recovery, and actually beginning to supply Mo-99 to hospitals. It is also too soon to know whether user-based incentives will yield the desired outcomes. At the international level, OECD NEA studies have argued in favor of leveling the playing field for new Mo-99 producers as well as the creation of a reserve capacity that would be "transparent and verifiable to ensure trust" for all.[63]
Despite substantial progress to date, success in balancing supply and security concerns is not assured. A "rosy" scenario depicts a future with an abundance of Mo-99, all of which is produced without the use of weapons-grade materials. However, a worst case scenario still involves a world with shortages of Mo-99 that continues to rely on exports of nuclear weapons-suitable materials. Thus, finding a timely solution to the dual challenge of making the Mo-99 supply chain reliable and HEU-free is critical.
Sources:
[1] The number is from John J. Szymanski and Parrish Staples, "Ensuring a Reliable Supply of Medical Radioisotopes," Office of Science and Technology Policy blog, March 27, 2012, www.whitehouse.gov.
[2] Radioisotopes are unstable forms of elements that emit radiation during their decay process. More than 100 different radioisotopes are used in various sectors of the U.S. economy today. See Jeff Norenberg, Parrish Staples, Robert Atcher, Robert Tribble, Jack Faught, and Lee Riedinger, "The Nation's Needs for Isotopes: Present and Future," summary of an August 2008 Department of Energy workshop, p. 29, science.energy.gov.
[3] The radioisotope is eluted from the Tc-99m generator using procedure-specific kits supplied by manufacturers.
[4] HEU is uranium which has been altered to the share of the fissionable isotope U-235 is greater than 20 percent. By contrast, natural uranium has less than 1 percent U-235 while low enriched uranium (LEU) has less than 20 percent U-235. HEU can be used to produce nuclear weapons; LEU cannot. Typically, weapons designers use HEU enriched to as much as 90 percent U-235. HEU is also seen as particularly vulnerable to terrorists because it can be used in an unsophisticated gun-type bomb like the Little Boy that destroyed Hiroshima; plutonium devices, by contrast, typically require more sophisticated implosion devices.
[5] The author wishes to thank Miles Pomper, Alan Kuperman, and Ferenc Dalnoki-Veress for their assistance with the preparation of this brief. All errors or omissions are her own.
[6] This tongue-in-cheek reference is from N. Ramamoorthy, "Production and Supplies of Mo-99: Lessons Learnt and New Options within Research Reactors and Neutron Sources Community," BARC, India, 2011, www.iaea.org.
[7] "About Nuclear Medicine & Molecular Imaging: Historical Timeline," Society of Nuclear Medicine and Molecular Imaging, undated, www.snm.org.
[8] See Advancing Nuclear Medicine Through Innovation, National Academy of Sciences, 2007, pp. 17-18.
[9] See surveys and projections in "The Supply of Medical Radioisotopes: An Assessment of Long-Term Demand for Technetium-99m," OECD Nuclear Energy Agency study, June 2011, www.oecd-nea.org.
[10] Joseph M. Kaminski, "Tc-99m Shortage," presentation at 2009 SNM Annual Meeting, June 2009, interactive.snm.org.
[11] Cristina Hansell, "Nuclear Medicine's Double Hazard," The Nonproliferation Review, Vol. 15, No. 2, July 2008, p. 193.
[12] Isotopes for the Nation's Future: A Long Range Plan, NSAC Isotopes Subcommittee report, 27 August 2009, p. 42, science.energy.gov.
[13] Isotopes for the Nation's Future: A Long Range Plan, NSAC Isotopes Subcommittee report, 27 August 2009, p. 42.
[14] Isotopes for the Nation's Future: A Long Range Plan, NSAC Isotopes Subcommittee report, 27 August 2009, p. 42, science.energy.gov.
[15] Isotopes for the Nation's Future: A Long Range Plan, NSAC Isotopes Subcommittee report, 27 August 2009, p. 42, science.energy.gov.
[16] The NRX reactor at Chalk River Laboratories was shuttered in 1993. On NRU conversion, see D.F. Sears and K.T. Conlon, "Development of LEU Fuel for Research Reactors: NRU, MAPLE, and SLOWPOKE," presentation in Oslo, Norway, June 2006, www.7ni.mfa.no.
[17] Cristina Hansell, "Nuclear Medicine's Double Hazard," The Nonproliferation Review, Vol. 15, No. 2, July 2008, p. 193.
[18] Isotopes for the Nation's Future: A Long Range Plan, NSAC Isotopes Subcommittee report, 27 August 2009, p. 42, science.energy.gov.
[19] Medical Isotope Production without Highly Enriched Uranium, National Academy of Sciences, 2009, p. 33. At present, this process is being investigated by B&W in its effort to set up domestic isotope production while a Russo-Canadian venture is again assessing the possibility of using the AHR at Kurchatov for Mo-99 production.
[20] The following reasons are cited for the shift to foreign suppliers for isotopes in general:: "the rising costs of operations at the U.S. national laboratories, DOE past pricing policy changes that resulted in substantial price increases that were unacceptable to the user community, parasitic operation [a situation in which the production of isotopes at a facility is not its "primary mission, but a byproduct of other activities] of facilities in the United States leading to irregular supply schedules, the age and unreliability of the equipment at national laboratories, retiring technical personnel, and the application of full cost recovery (Public Law 101) to the cost of 'commercial' isotopes." See Jeff Norenberg, Parrish Staples, Robert Atcher, Robert Tribble, Jack Faught, and Lee Riedinger, "The Nation's Needs for Isotopes: Present and Future," summary of an August 2008 Department of Energy workshop, p. 28.
[21] This is a reference to a Society of Nuclear Medicine awareness campaign by that title. See https://interactive.snm.org.
[22] See the video "Logistics of Lifesaving: Two Days in the Life of Mo-99," MDS Nordion, undated, www.nordion.com.
[23] "White Paper: Transporting Radiopharmaceuticals in the United States," CORAR, October 2010, www.corar.org.
[24] Using passengers aircraft for the transportation of radioactive materials poses its own challenges. See "Breaking Bottlenecks: IAEA Tackles Transport Woes of Life-saving Radioactive Materials," IAEA staff report, January 25, 2011, www.iaea.org.
[25] 25 kg of U-235 HEU constitutes a significant quantity of HEU (e.i. enough for one bomb). The 45 kg number is from N. Ramamoorthy, "Production and Supplies of Mo-99: Lessons Learnt and New Options within Research Reactors and Neutron Sources Community," BARC, India, 2011, www.iaea.org.
[26] Medical Isotope Production without Highly Enriched Uranium, National Academy of Sciences, 2009, p. 35.
[27] Medical Isotope Production without Highly Enriched Uranium, National Academy of Sciences, 2009, p. 35.
[28] See, for example, F. Dalnoki-Veress, C. Hansell, M. Pomper, "An Evaluation of Mo-99 Target Waste Proliferation Risks," paper presented at 2009 RERTR meeting in Beijing, China, November 2009, www.rertr.anl.gov.
[29] Cristina Hansell, "Nuclear Medicine's Double Hazard," The Nonproliferation Review, Vol. 15, No. 2, July 2008, pp. 193-194.
[30] Medical Isotope Production without Highly Enriched Uranium, National Academy of Sciences, 2009, p. 18.
[31] Because of this, the Tc-99m/Mo-99 generators are priced in so-called 6-day curies (6-day Ci). Medical Isotope Production without Highly Enriched Uranium, National Academy of Sciences, 2009, p. 35. This unit refer to the number of curies (Ci) that remain in a Mo-99 shipment six days after it leaves the processing facility.
[32] This is known as eluting or "milking" the generator. See Medical Isotope Production without Highly Enriched Uranium, National Academy of Sciences, 2009, p. 18.
[33] The BR-2 ran on HEU fuel while the SAFARI was in the process of converting to the use of both LEU fuel and targets.
[34] "The Supply of Medical Radioisotopes: An Assessment of Long-Term Demand for Technetium-99m," OECD Nuclear Energy Agency study, June 2011, www.oecd-nea.org, p. 8.
[35] AJ Kuperman, "The Global Threat Reduction Initiative and Conversion of Isotope Production to LEU Targets," paper presented at the 2004 RERTR Conference in Vienna, Austria, November 2004.
[36] AJ Kuperman, "The Global Threat Reduction Initiative and Conversion of Isotope Production to LEU Targets," paper presented at the 2004 RERTR Conference in Vienna, Austria, November 2004.
[37] Medical Isotope Production without Highly Enriched Uranium, National Academy of Sciences, 2009, p. 2.
[38] Medical Isotope Production without Highly Enriched Uranium, National Academy of Sciences, 2009, pp. 5-6.
[39] Parrish Staples, "DOE/NNSA Efforts to Support the Domestic Production of Mo-99," 11 May 2012, NRC hearing.
[40] Jeff Chamberlin, "The Role of International Cooperation Programs in International HEU Minimization," presentation at the Second International Symposium on HEU Minimization in Vienna, Austria, January 2012.
[41] Todd Jacobson and Sarah Herness, "NNSA to Reconsider How to Jumpstart Isotope Industry," Nuclear Weapons & Materials Monitor, February 10, 2012, pp. 8-10.
[42] See, for example, Matthew L. Wald, "Radioisotope Recipe Lacks One Ingredient: Cash," New York Times, February 6, 2012.
[43] "Record Levels of Non-HEU-Based Mo-99 Supplied to the United States," National Nuclear Security Administration press release, June 2, 2011, nnsa.energy.gov.
[44] See "CRP on Production of Mo-99 from LEU or Neutron Activation," IAEA Research Reactor Section, undated, www.iaea.org.
[45] "Joint Statement on Quadrilateral Cooperation on High-density Low-enriched Uranium Fuel Production," March 27, 2012, press release available at: www.nnsa.energy.gov.
[46] AJ Kuperman, "The Global Threat Reduction Initiative and Conversion of Isotope Production to LEU Targets," paper presented at the 2004 RERTR Conference in Vienna, Austria, November 2004.
[47] The far lower level of the fissile isotope U-235 in LEU would lead to fewer fission reactions and a subsequent flow of neutrons. And that in turn would produce less Mo-99 per target. The U.S. government in the 1980s developed and qualified a fuel design which packed more uranium into a target but the increased uranium density of this uranium silicide dispersion fuel was still not sufficient to make up for all of the difference between HEU and LEU.
[48] Miles Pomper, ""HEU Minimization after the Seoul Nuclear Security Summit," paper presented at the International Nuclear Materials Management conference in Orlando, Florida, July 2012.
[49] Ron Cameron and Chad Westmacott, "OECD-Nuclear Energy Agency's Policy Approach for a Reliable Supply of Mo-99," paper presented at the Mo-99 Topical Meeting in Santa Fe, New Mexico, December 2011, p. 7.
[50] Ron Cameron and Chad Westmacott, "OECD-Nuclear Energy Agency's Policy Approach for a Reliable Supply of Mo-99," paper presented at the Mo-99 Topical Meeting in Santa Fe, New Mexico, December 2011, p. 7.
[51] Miles Pomper, ""HEU Minimization after the Seoul Nuclear Security Summit," paper presented at the International Nuclear Materials Management conference in Orlando, Florida, July 2012.
[52] Miles Pomper, ""HEU Minimization after the Seoul Nuclear Security Summit," paper presented at the International Nuclear Materials Management conference in Orlando, Florida, July 2012.
[53] Kendra Vessels, "HEU for Isotope Production in Canada and Russia: Expansion or Phaseout?," University of Texas in Austin paper, April 20, 2011, www.heuphaseout.org
[54] "A Supply and Demand Update of the Molybdenum-99 Market," NEA OECD, August 2012, www.oecd-nea.org.
[55] "Markey, Fortenberry Call on Obama to Halt Russian Production of Medical Isotopes Using Highly Enriched Uranium," press release, May 5, 2011, https://markey.house.gov.
[56] "Public Health and Nuclear Experts Warn Against Importing Russian Medical Isotopes," press release by the Nuclear Proliferation Prevention Project, January 18, 2012, available at blogs.utexas.edu.
[57] Douglas Guarino, "Suggestions Russia May Limit HEU Use Prompts Cautious U.S. Response," Global Security Newswire, August 17, 2012, www.nti.org.
[58] "Encouraging Reliable Supplies of Molybdenum-99 Produced without Highly Enriched Uranium," The White House Office of the Press Secretary, June 7, 2012, www.whitehouse.gov.
[59] "Encouraging Reliable Supplies of Molybdenum-99 Produced without Highly Enriched Uranium," The White House Office of the Press Secretary, June 7, 2012, www.whitehouse.gov.
[60] "Encouraging Reliable Supplies of Molybdenum-99 Produced without Highly Enriched Uranium," The White House Office of the Press Secretary, June 7, 2011, www.whitehouse.gov.
[61] Douglas P. Guarino, "U.S. Proposes Bigger Medicare Payouts to Avoid Bomb-Grade Uranium," Global Security Newswire, July 11, 2012, www.nti.org.
[62] "Encouraging Reliable Supplies of Molybdenum-99 Produced without Highly Enriched Uranium," The White House Office of the Press Secretary, June 7, 2012, www.whitehouse.gov.
[63] Ron Cameron and Chad Westmacott, "OECD-Nuclear Energy Agency's Policy Approach for a Reliable Supply of Mo-99," paper presented at the Mo-99 Topical Meeting in Santa Fe, New Mexico, December 2011, p. 7.
[64] Inspired by image in Ira Goldman, "Toward a More Secure Future?" available at nuclearfoundation.org
Sign up for our newsletter to get the latest on nuclear and biological threats.
The NTI Index is recognized as the premier resource and tool for evaluating global nuclear and radiological security.
Nuclear and radiological security aims to ensure nuclear and other radioactive materials are secure from unauthorized access and theft, and that nuclear facilities are secure from sabotage.
The under-the-radar success story of U.S.-Chinese cooperation to convert reactors from HEU to LEU to reduce proliferation and nuclear terrorism risks. (CNS)