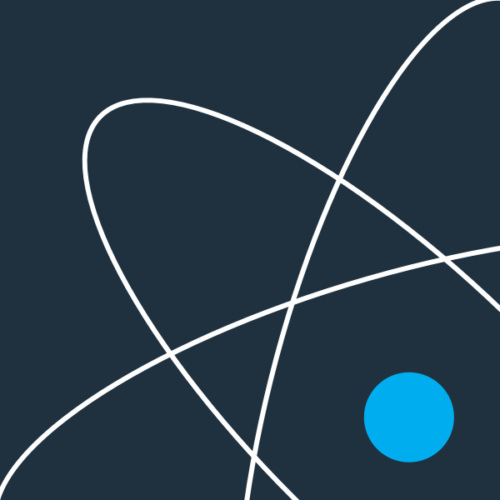
Charles D. Ferguson
Scientific Consultant, Fellow for Science and Technology, The James Martin Center for Nonproliferation Studies
Fifty years ago, commercial nuclear power production was launched. Around that time, nuclear industry analysts projected shortages of uranium fuel occurring in the near term based on predictions of nuclear power plant production. To provide fuel for future nuclear reactors, many nations invested significant resources into reprocessing of spent nuclear fuel to extract plutonium and to recycle uranium. In 1977, the U.S. government decided against pursuing reprocessing because of concerns that encouraging plutonium separation from spent fuel could promote nuclear proliferation. Moreover, the steep financial costs of reprocessing argued against development of a plutonium economy. During the mid- to late 1970s, orders for new nuclear plants stagnated, further reducing the demand for uranium-based fuels and plutonium reprocessing. Presently, the world produces about 16% of its electricity from nuclear energy.
Today, there is renewed interest in the expansion of nuclear energy in order to meet the world’s energy needs and to lesson the impact of global warming due to emission of greenhouse gases from fossil fuels, such as petroleum. However, in addition to impediments to siting repositories for radioactive waste, the global problems of proliferation and the threat of nuclear terrorism act as countervailing forces to development of a plutonium economy to support increases in nuclear power. Growing stockpiles of separated civilian plutonium increase the risk that states or terrorists might acquire this material to build nuclear weapons. Public health and reactor safety could also worsen if the use of plutonium-fuel in commercial power plants expands beyond the current small level of usage. Research and development of advanced proliferation-resistant fuel cycles and safer reactor designs may eventually lead to greater use of plutonium in power plants. The continuing high economic costs associated with these programs, however, will likely slow adoption.
On June 27, 2004, the world celebrated the 50th anniversary of commercial nuclear power. Fifty years ago, the Obninsk nuclear power station in Russia was commissioned, helping to spark the growth of civilian nuclear energy. This expansion lasted into the mid- to late 1970s when orders for new nuclear plants stagnated in the United States and have yet to recover although the nuclear industry touts signs of a nuclear renaissance. The United States still has 103 operating commercial reactors, the largest number of any other nation. Today, about 440 civilian reactors are generating about 16% of the world's electricity.[1]
During the golden age of nuclear power from the 1950s to the late 1970s, nuclear industry leaders envisioned building even more reactors than currently exist. At that time, dreams of nuclear electricity "too cheap to meter" notwithstanding, nuclear fuel projections pointed toward potential shortages of uranium in the near term. Only 0.7% of natural uranium is uranium-235, the form, or isotope, of uranium used to fuel most nuclear reactors. The most abundant isotope, uranium-238, which comprises 99.2% of natural uranium, does not readily fission. Plutonium production appeared to offer a solution to projected shortfalls of uranium fuel.
As indicated, both uranium and plutonium can fuel reactors to release large amounts of nuclear energy, which can be transformed into electricity. Because plutonium isotopes decay much more rapidly than uranium isotopes, uranium is far more naturally abundant than plutonium, which exists in only trace quantities in nature. Nuclear reactors, however, make plutonium when uranium-238 absorbs neutrons and then undergoes a relatively rapid series of radioactive decays to become plutonium-239. Thus, the relatively huge amounts of uranium-238 available in mines located in many nations could, in principle, serve as fertile material to produce vast amounts of nuclear fuel.
Plutonium-239, as well as other plutonium isotopes, easily fissions and contributes up to 40% of the energy released in a typical light-water reactor that is initially fueled with uranium. Three-fourths (about 350) of the world's commercial reactors are light-water reactors in which ordinary water cools the reactor's core by transporting nuclear energy from the core to the part of the plant that produces electricity. Water also moderates, or slows down, neutrons in order to increase the likelihood of causing uranium-235 and plutonium-239 to fission and emit energy.
Nuclear fuel in a light-water reactor lasts typically one to three years before the buildup of radioactive fission products makes it increasingly harder to extract energy from a fuel assembly. At this point, the plant operators remove the spent fuel and then add fresh fuel to the reactor core. The spent fuel, however, still contains uranium-235 and various isotopes of plutonium. This material could potentially be used to create more energy. A technique known as reprocessing can chemically separate the uranium and plutonium from the other radioactive materials in spent fuel. By weight percentage, spent fuel typically consists of 95.6% uranium (with most of that being uranium-238), 3% stable or short-lived radioactive fission products, 0.3% cesium and strontium (the primary sources of high-level radioactive waste over a few hundred years), 0.1% long-lived iodine and technetium, 0.1% long-lived actinides (heavy radioactive elements), and 0.9% plutonium.
The uranium and plutonium can be recycled into new fuel. Doing this is called closing the nuclear fuel cycle. In contrast, an open fuel cycle, also known as a once-through cycle, would use the initial batch of uranium fuel once and then dispose of the spent fuel, rather than extracting the unused uranium and plutonium.
Because of the aforementioned uranium shortage projections, many nations invested in reprocessing methods to provide a source of recycled fuel. As a result, reprocessing knowledge and technology spread widely across the world. The plutonium extracted by reprocessing could be formed into fuel for reactors employing slow, or thermal, energy neutrons, such as light-water reactors, or could be used to power a different type of reactor: the fast neutron breeder reactor. The uranium shortfall forecasts spurred intense interest in developing fast breeder reactors to create, or breed, new fuel and concurrently burn plutonium. Making use of the principle mentioned above, that uranium-238 can absorb neutrons to produce plutonium, these reactors, which are called "fast" because they employ fast, or high energy, neutrons to fission fuel, can "breed" more fuel than they consume. Thus, the projected limited supply of uranium could allow a bootstrapping to a plutonium economy through large-scale use of fast breeder reactors.
The nuclear ploughshare offered by reprocessing to close the fuel cycle, however, leads a double-life as a nuclear sword. The same technology can allow a nation to extract plutonium for use in nuclear bombs. In 1974, the "peaceful" nuclear explosion in India served as a wake-up call to the dangers of the proliferation of reprocessing methods. India had produced plutonium in the Cirus heavy-water research reactor (which used heavy water, rather than light water, as coolant and moderator for the reactor core). Canada had provided the reactor, and the United States had supplied the initial batch of heavy water. Through the dispersion of reprocessing knowledge stemming from U.S. President Dwight Eisenhower's Atoms for Peace program, which began in December 1954, India was able to acquire all that it needed to create and extract plutonium from a modestly sized reactor.
India's nuclear test provoked a reversal of U.S. government policy concerning plutonium reprocessing. Until 1977, the United States had supported closing the nuclear fuel cycle. In April of that year, then-President Jimmy Carter decided to defer indefinitely commercial reprocessing of plutonium. He also sought to persuade other nations to reconsider their commitments to reprocessing on the grounds of preventing nuclear proliferation and saving money. An important venue sponsored by the United States to broadcast this message was the International Nuclear Fuel Cycle Evaluation (INFCE) Conference, but opposition from France and the United Kingdom, in particular, which had invested substantial resources into reprocessing plants, stopped the INFCE from gaining traction.
In 1993, the Clinton administration reiterated this 1977 U.S. government policy by stating that "the U.S. does not encourage the civil use of plutonium and, accordingly, does not itself engage in plutonium reprocessing for either nuclear power or nuclear explosive purposes."[2] (The Ford Administration laid the groundwork for the Carter decision. Just prior to the 1976 U.S. presidential election, President Ford ordered a hold on the start of a new reprocessing plant in Barnwell, South Carolina, while safeguards issues were being examined.) While the Bush administration still adheres to this policy, it has expressed strong interest in proliferation-resistant methods of reprocessing, as discussed later.
As suggested above, economics played a role in contributing to Carter's decision. In particular, by the mid- to late 1970s, the nuclear industry experienced a major downturn in the demand for new nuclear power plants in the United States, and there was a realization that uranium supplies were not going to run out in the coming decades. Thus, reprocessing was and remains a more expensive endeavor than using uranium-based fuels in a once-through cycle. Nonetheless, the "energy crisis" during the 1973 Organization of Petroleum Exporting Countries (OPEC) oil embargo sounded an alarm about the urgent need to invest in alternative energy resources instead of relying heavily on fossil fuels, such as petroleum and natural gas. Japan, for instance, around that time began investing significant resources into a pilot-scale reprocessing plant at Tokai-mura. Development of a commercial-scale plant at Rokkasho-mura proceeded gradually as Japan tapped into the reprocessing capabilities available in Europe. France and the United Kingdom built large reprocessing facilities at La Hague and Sellafield, respectively.
Despite the U.S. decision to halt plutonium reprocessing, these industrialized countries, including Russia at the Mayak RT-1 facility, have continued to separate plutonium from spent fuel. Japan has yet to commission its commercial-scale plant, but Rokkasho-mura, when completed, could reprocess about 800 metric tons of spent fuel annually yielding approximately seven metric tons of separated plutonium.
Reprocessing activities, to date, have accumulated a large stockpile of civilian plutonium. In particular, reprocessing plants have separated more than 200 metric tons; of that, more than 175 tons remain in storage waiting to be used in nuclear power plants. These plutonium stocks would probably have grown much larger if the United States had continued to encourage separation of plutonium. For comparison, worldwide use of nuclear power has, to date, amassed more than 1,000 metric tons of plutonium in spent nuclear fuel. Conceivably, if reprocessed, this spent fuel could more than quintuple the amount of separated civilian plutonium. The civilian plutonium stockpile is somewhat less than the estimated military plutonium inventory of about 250 metric tons. At current trends of plutonium separation and use, the civilian stocks will exceed the military supply within the next 20 years.
Demand has not kept up with stockpiling of plutonium. The small demand is mainly concentrated in Europe. In particular, some 30 reactors in the countries of Belgium, Switzerland, Germany, and France use mixed oxide (MOX) fuel in light-water reactors. (MOX consists mainly of depleted uranium, which contains less uranium-235 than natural uranium, and from four to seven percent plutonium. The uranium and plutonium in MOX are chemically combined with oxygen.) Germany plans to phase out its nuclear power plants, however.
In East Asia, use of MOX may yet expand. Japan has ambitious plans to employ MOX in a third of its commercial reactors by 2010, but local governments there tend to look unfavorably on this proposal. In late 2003, China expressed interest in buying the Siemens MOX fuel production plant, which was originally scheduled to be constructed in Hanau, Germany. Even factoring in this proposed usage of MOX, the rate of accumulation of civilian plutonium will likely continue to exceed the rate of consumption. Presently, less than 10 percent of the world's commercial nuclear power plants burn MOX fuel.
Light-water reactors are not ideal for plutonium burning. However, earlier plans to build a fleet of plutonium-consuming and plutonium-producing fast breeder reactors have floundered. Both the French Superphénix and Japanese Monju fast reactors experienced safety problems, forcing their shutdown. Moreover, these types of reactors are usually more expensive to operate than reactor designs relying on well-proven slow, or thermal, neutron technologies. Only Russia with its operational BN-600 fast reactor still harbors serious hopes of building a large number of fast reactors, as discussed in more detail later. The BN-600 is the sole commercial fast reactor in use in the world. Russia has resumed construction of a larger version, the BN-800. In addition, India and Japan have plans for commercializing fast reactors but will likely continue to confront roadblocks.
While not the focus of this Issue Brief, usage of weapons-grade plutonium in MOX fuel resulting from the American and Russian Plutonium Disposition Program would substantially increase the commerce in this fuel. For now, the program remains stalled because of inadequate donor support for the Russian part of the project and a festering dispute over who will cover liability in the case of an accident.
Are the security risks of civilian plutonium overblown? Some have argued that because this material is not "weapons-grade," the proliferation risks are minimal.[3] While nations that seek nuclear weapons would prefer weapons-grade material, reactor-grade plutonium could also power an explosive chain reaction. During the long irradiation time in a typical commercial power plant, plutonium continues to absorb neutrons, transforming the ideal bomb material plutonium-239 (Pu-239) into other isotopes, including Pu-240, Pu-241, and Pu-242. Weapons-grade plutonium contains less than 6 percent of Pu-240 and much smaller percentages of Pu-238, Pu-240, and Pu-241.
The non-ideal isotopes are such because they emit more neutrons through spontaneous fission and release more heat than Pu-239. These effects can complicate weapons design. Although the greater heat emissions from reactor-grade material would complicate the engineering of the weapon, this problem is surmountable.[4] In 1962, the United States reportedly detonated an explosive device made from plutonium that was less than weapons-grade; however, the exact isotopic composition has not been openly published.
The excess neutrons significantly increase the likelihood of a "fizzle" bomb, a weapon that would prematurely detonate and would thus produce a smaller explosive yield than a bomb made from more reliable weapons-grade material. Nonetheless, the important point is that the reactor-grade bomb is almost guaranteed to produce an explosive yield, as long as other design features are done correctly. The typical "fizzle" yield would be about one kiloton, which would "have a radius of destruction roughly one-third that of the Hiroshima weapon, making it a potentially fearsome explosive. Regardless of how high the concentration of troublesome isotopes is, the yield would not be less," according to a report by the National Academy of Sciences.[5]
Although states would not be inclined to use reactor-grade plutonium because the explosive yield would be unreliable from a military standpoint, terrorists seeking to build and detonate improvised nuclear devices would probably just be interested in creating any yield. Their main objective with a nuclear terror attack would be to terrorize the public. Even fizzle yields would strike fear in citizens as well as cause massive destruction.
Plutonium is not the prime choice of nuclear terrorists. Terrorists would prefer to acquire the easiest nuclear materials to turn into a nuclear bomb. Highly enriched uranium (HEU) tops the ease-of-use hierarchy. Unlike plutonium, HEU can be used in the simplest type of nuclear weapon, a gun-type device.[6] If nuclear terrorists cannot acquire HEU, they would then try to seize plutonium, which would have to be used in the more technically challenging implosion-type device. Weapons-grade plutonium would obviously be the most desirable form of plutonium, but if terrorists only had access to reactor-grade material, they would most likely try to use it.[7]
Growing stocks of separated civilian plutonium increase the risk that terrorists could seize this weapons-usable material. In light of increased perceived threats of nuclear terrorism, states that continue to separate plutonium from spent fuel should reconsider this activity or at least re-evaluate the security around reprocessing plants.
In addition to possible plutonium seizure or diversion from reprocessing sites, increasing commerce in plutonium and plutonium-bearing fuels, such as MOX fuel, raises the targets of opportunity for terrorists. If terrorists acquired MOX, they could attempt to separate the plutonium from the depleted uranium using well-known chemical techniques. Nevertheless, such capabilities would likely only be accessible to well-financed and well-organized terrorist organizations able to recruit or hire the requisite technical talent.
On the state level, as mentioned above, weapons-grade plutonium is also preferred. However, if a state wanted to use stocks of civilian reactor-grade plutonium, it could. According to the National Academy of Sciences, "In short, it would be quite possible for a potential proliferator to make a nuclear explosive from reactor-grade plutonium using a simple design that would be assured of having a yield in the range of one to a few kilotons, and more using an advanced design. Theft of separated plutonium, whether weapons-grade or reactor-grade, would pose a grave security risk."[8]
In addition to concerns about nuclear terrorism with improvised nuclear devices, the post-9/11 security environment has renewed attention to radiological terrorism. Even a dud plutonium bomb would still make a frightening and damaging radiological dispersal device, or "dirty bomb." Moreover, there are other ways to disperse radioactivity. Reprocessing plants contain huge quantities of highly radioactive waste. These and other locations at these plants, including spent fuel pools, processing lines, powdered plutonium oxide storage sites, could pose tempting targets for terrorist attack or sabotage, aiming to release radiation to harm public health and contaminate valuable property. In Sellafield, for instance, 21 steel tanks contain high-level radioactive waste holding about 211 megacuries (MCi) of cesium-137. In comparison, the 1986 Chernobyl accident released about 2.4 MCi of cesium-137. This is not to say that an attack on Sellafield would release its entire accumulation of radioactive material. However, even if one tank were ruptured, this event could cause serious contamination, which, at a minimum, would result in substantial financial costs.
Can the world use plutonium fuel in nuclear reactors while reducing the proliferation risks? Both the United States and Russia subscribe to the policy of proliferation-resistance in the nuclear fuel cycle. In 2000, the Russian Federation published its vision for nuclear energy in the 21st century. This policy statement made clear Russia's view that plutonium is a valuable resource. It stated, "Plutonium, as a nuclear material, has unique energy potential, is nationally owned, and can be put to maximum effective use under Russia's national energy strategy." Moreover, "Russia's nuclear fuel strategy is based on the concept of a closed fuel cycle and the establishment of corresponding production capacity and technology."[9]
Russia is focused on capitalizing on fast breeder reactor technology. Notably, the Russian statement drops the term "breeder," which has negative connotations associated with producing vast stockpiles of plutonium. The new breed of fast reactors would be designed to burn plutonium without using a uranium blanket to create huge quantities of new plutonium. Nonetheless, a seriou investment in and expansion of fast reactors, whether as breeders or not, could spur a large-scale plutonium economy with increased shipments of plutonium-fuel throughout Russia and other parts of the world.
Although not as forward leaning as Russia on the focus on closed fuel cycles and fast reactors, the Bush administration in its National Energy Policy supported a renewal and expansion of nuclear power and called for a reconsideration of past government policies, such as the 1977 Carter and 1993 Clinton administrations' ban on plutonium reprocessing as long as plutonium is not made bomb-usable. In particular, "The NEPD Group recommends that, in the context of developing advanced nuclear fuel cycles and next generation technologies for nuclear energy, the United States should reexamine its policies to allow for research, development and deployment of fuel conditioning methods (such as pyroprocessing) that reduce waste streams and enhance proliferation resistance. In doing so, the United States will continue to discourage the accumulation of separated plutonium, worldwide."[10]
Pyroprocessing is touted by many nuclear energy enthusiasts as a way to use plutonium as fuel without increasing the risks of proliferation.[11] In principle, this method would keep a highly radioactive fission product barrier around the plutonium. Such a barrier would deter thieves and terrorists from seizing the plutonium because they could receive a lethal dose if the radioactive material is unshielded.
If it were the only reprocessing technique in use, pyroprocessing would not reduce the risk of proliferation to zero. Nations trying to obtain the bomb could operate a clandestine PUREX reprocessing plant in parallel to the pyroprocessing facility. PUREX stands for plutonium-uranium reduction extraction and as the name implies, this method extracts or separates plutonium from the highly radioactive components of spent fuel. It is presently employed in all commercial reprocessing plants. The knowledge of this technique is widespread. Thousands of people throughout the world are familiar with the chemistry and engineering required for PUREX.
Consequently, rigorous safeguards to protect against diversion of plutonium into weapons programs would continue to be essential in a future consisting of pyroprocessing plants. In 2003, the MIT Nuclear Energy Study Advisory Committee, which included several former U.S. government officials, examined the proliferation risks of once-through fuel cycles, traditional closed cycles, and advanced fuel cycles, such as those employing pyroprocessing. This committee concluded, "In summary, the global growth scenario built primarily upon the once-through thermal reactor fuel cycle would sustain an acceptable level of proliferation resistance if combined with strong safeguards and security measures and timely implementation of long term geological isolation. The PUREX/MOX fuel cycle produces separated plutonium and, given the absence of compelling reasons for its pursuit, should be strongly discouraged in the growth scenario on nonproliferation grounds. Advanced fuel cycles may achieve a reasonable degree of proliferation resistance, but their development needs constant evaluation so as to minimize risk."[12]
The U.S. National Energy Policy, which was written under the leadership of Vice President Dick Cheney, also encouraged cooperation with other nations in the expansion of nuclear energy. It stated, "The United States should also consider technologies, in collaboration with international partners with highly developed fuel cycles and a record of close cooperation, to develop reprocessing and fuel treatment technologies that are cleaner, more efficient, less waste-intensive, and more proliferation-resistant." For instance, the United States has had a cooperative nuclear energy program with Russia.
International cooperation is facilitated by transparency of nuclear activities. In 1997, nine countries (China, France, Russia, the United Kingdom, and the United States — the five nuclear weapon states – in addition to Japan, Germany, Belgium, and Switzerland) agreed to the Guidelines for the Management of Plutonium, codified as INFCIRC/549, which is published by the International Atomic Energy Agency.[13] They pledged to issue occasional statements of their holdings of civil plutonium in unirradiated form and in spent nuclear fuel. If continually strengthened by regular and frequent statements about plutonium stockpiles by these states, this framework could support improved nuclear safeguards. The 2003 MIT nuclear energy study specified that "The IAEA safeguards framework should move from an approach based on accounting/reporting and periodic inspection to an approach based on continuous surveillance/containment/security. This is crucial for PUREX/MOX fuel cycle facilities."[14]
Safeguards are not foolproof. During normal reprocessing plant use, some plutonium will be lost in production. Even small accounting errors could mask the diversion of plutonium. For example, while not implying that Japan has diverted plutonium, accounting uncertainties at a plant the size of Rokkasho-mura could easily exceed a significant quantity: 8 kg of plutonium. (The IAEA deems a significant quantity enough material to make a first-generation nuclear bomb.) Although Japan has been one of the most examined countries by the IAEA, there have been accounting discrepancies that have raised concern among some.
In addition to proliferation concerns, plutonium-fueled reactors could increase the risk of nuclear accidents. If such an accident occurred and released radioactive materials, public health could be at greater danger from plutonium-fueled reactors rather than from uranium-fueled power plants because of the greater health hazards associated with plutonium.[15]
Nothing lasts forever, including nuclear power plants. After many years of neutron bombardment, reactor vessels become brittle. According to David Lochbaum, a nuclear engineer with the Union of Concerned Scientists, "More plutonium atoms will be split in MOX-fueled plants than in non-MOX-fueled plants. The splitting of plutonium atoms releases more neutrons at higher energy levels than the splitting of uranium atoms. More neutrons traveling at greater speeds may increase the number that strike the metal reactor vessel containing the reactor core. Neutrons weaken metal by making it more brittle."[16]
Nuclear plant managers like to point out that their plants incorporate defense-in-depth safety systems. If one system fails, a redundant one will take its place to mitigate the likelihood of a major accident. This safety setup is almost correct. The major gap is the reactor vessel. If it fails, there is no backup. A containment structure around the core may prevent a major release of radioactivity, but at a minimum, the core will be destroyed if the vessel shatters, resulting in substantial economic losses.
A new generation of reactors could be designed to burn plutonium fuel with vessels constructed to withstand the rigors of increased neutron bombardment. Concerning the current generation, nuclear regulatory agencies take into account the potential for vessel failure and accordingly do not allow most of the core to be loaded with MOX fuel. The typical MOX loading is one-third of the core. While this fuel configuration reduces the chances of an accident, it significantly limits the amount of plutonium that can be burned in the current types of reactors.
Even if the security and safety risks could be reduced to acceptable levels of concern, the financial cost of plutonium reprocessing would likely remain prohibitive for a long time to come. In December 2003, a Harvard University research group, funded by the Department of Energy, calculated the break-even point when reprocessing would become competitive with the once-through fuel cycle. This study found, "At a reprocessing price of $1000 per kilogram of heavy metal (kgHM), and with our other central estimates for the key fuel cycle parameters, reprocessing and recycling plutonium in existing light-water reactors (LWRs) will be more expensive than direct disposal of spent fuel until the uranium price reaches over $360 per kilogram of uranium (kgU) – a price that is not likely to be seen for many decades, if then."[17] The group noted that the $1000 per kgHM assumption is a conservative cost estimate, "which is substantially below the cost that would pertain in privately financed facilities with identical costs and capacities to the large commercial facilities now in operation."
That report also examined the economics of closed fuel cycles and fast reactors compared to the once-through cycle and concluded, "Reprocessing and recycling plutonium in fast-neutron reactors (FRs) with an additional capital cost, compared to new LWRs, of $200/kWe [kWe equals kilowatts of electric power] installed will not be economically competitive with a once-through cycle in LWRs until the price of uranium reaches some $340/kgU … Even if the capital cost of new FRs could be reduced to equal that of new LWRs, recycling in FRs would not be economic until the uranium price reached some $140/kgU." Uranium market prices are now around $80/kgU and have not increased in a couple of decades. They are not expected to increase significantly in the coming years.
Although economics should not be the only driver in determining whether plutonium reprocessing and recycling is worth investing in, the continuing real risks that states or terrorists seeking the bomb can acquire civilian plutonium argue against moving forward with a plutonium economy. Pyroprocessing, advanced fuel cycles, and development of a proliferation-resistant generation of reactors may yet usher in a new nuclear golden age. Like the first 50 years of commercial nuclear power, the next 50 years will most certainly be a momentous time, however hard to predict.
[1] World Nuclear Association, "World Nuclear Power Reactors 2003-2004 and Uranium Requirements," June 26, 2004, www.world-nuclear.org.
[2] White House Fact Sheet, "Nonproliferation and Export Control Policy Statement," September 27, 1993.
[3] See, for example, Bruno Pellaud, "Proliferation Aspects of Plutonium Recycling," Journal of Nuclear Materials Management, Fall 2002, pp. 30-38, www.inmm.org.
[4] J. Carson Mark, "Explosive Properties of Reactor-Grade Plutonium," Science & Global Security, Volume 4, 1993, pp. 111-128, www.princeton.edu; Richard L. Garwin, "Reactor-Grade Plutonium can be used to Make Powerful and Reliable Nuclear Weapons: Separated plutonium in the fuel cycle must be protected as if it were nuclear weapons," Presentation, August 26, 1998, www.fas.org.
[5] Committee on International Security and Arms Control, National Academy of Sciences, Management and Disposition of Excess Weapons Plutonium, National Academy Press, Washington, DC, 1994, p. 33.
[6] There is an urgent need for governments to adopt an HEU First Strategy, which would secure, consolidate, and eliminate HEU stocks as quickly as possible. See the recommendations in Charles D. Ferguson and William C. Potter with Amy Sands, Leonard S. Spector, and Fred L. Wehling, The Four Faces of Nuclear Terrorism (Monterey Institute of International Studies and the Nuclear Threat Initiative, 2004), www.nti.org.
[7] J. Carson Mark, "Reactor-Grade Plutonium's Explosive Properties," Report for the Nuclear Control Institute, August 1990, www.nci.org.
[8] Committee on International Security and Arms Control, National Academy of Sciences, Management and Disposition of Excess Weapons Plutonium, p. 33.
[9] Ministry of the Russian Federation for Atomic Energy, Strategy of Nuclear Power Development in Russia in the First Half of the 21st Century, 2000, https://old.minatom.ru.
[10] National Energy Policy, The White House, Washington, DC, May 2001, www.whitehouse.gov.
[11] See, for example, William H. Hannum, Gerald E. Marsh, and George S. Stanford, "PUREX and Pyro are not the same," Physics & Society, July 2004, www.aps.org.
[12] MIT Nuclear Energy Study Advisory Committee, The Future of Nuclear Power, Massachusetts Institute of Technology, 2003, https://web.mit.edu.
[13] International Atomic Energy Agency, "Guidelines for the Management of Plutonium," INFCIRC/549 Documents, 1997 to present, www.iaea.or.at.
[14] MIT Nuclear Energy Study Advisory Committee, The Future of Nuclear Power, p. 88.
[15] Edwin S. Lyman, "Public Health Risks of Substituting Mixed-Oxide for Uranium Fuel in Pressurized-Water Reactors," Science & Global Security, Volume 9, 2001, pp. 33-79, www.princeton.edu.
[16] David Lochbaum, "Reactor Operation with MOX Fuel: Real Use Crying About Spoiled Milk," Presentation at "People's Forum on Plutonium Fuels," November 9, 2000, www.ucsusa.org.
[17] Matthew Bunn, Steve Fetter, John P. Holdren, and Bob van der Zwaan, "The Economics of Reprocessing vs. Direct Disposal of Spent Nuclear Fuel," Project on Managing the Atom, Belfer Center for Science and International Affairs, December 2003, https://bcsia.ksg.harvard.edu.
Sign up for our newsletter to get the latest on nuclear and biological threats.
The co-conveners and participants of the Euro-Atlantic Security Leadership Group call for the re-establishment of basic principles relating to security and nuclear order.
This paper highlights the need for renewed attention to the catastrophic effects of nuclear conflict as a crucial step toward reducing the risk of nuclear use.
There is a critical need for a global diplomatic approach to address growing cyber risks, including, where possible, through cooperation between the United States and Russia.